Low-background radioassay facilities
Rare-event search experiments such as those searching for dark matter in our galaxy or evidence of neutrinoless double beta decay are typically deployed deep underground to shield them from cosmic-rays and other radiation present at the Earth's surface that would swamp the faint signals from new physics. However, they must also be constructed from extrememly radiologically pure materials for the same reason. Trace amounts of radiation present in every day materials, with emission of gamma-rays, neutrons, alpha-particles and radon, though harmless to us limit experimental sensitivity. Construction of the leading most sensitive dark matter experiments drives scientists to seek out the most radiopure materials known to humankind and often to push past this and develop entirely new techniques to remove residual radiological contaminants even further. In either case, it is crucial to measure and quantify the radiation from every single material and component that might go into constructing a detector. It is only by keeping the background from emission sufficiently low that we can hope to expose a small, rare signal. In addition, we must characterise the residual backgrounds accurately. It is only by having an incredibly precise background model that we can ascribe statisical significance to possible signal exceeding our background expectations.
The radioisotopes of particular concern contributing to backgrounds in lo-wbackground experiments are the gamma-ray emitting isotopes 40K; 137Cs; and 60Co, as well as 238U, 235U, 232Th, and their progeny. The U and Th chains are also responsible for neutron production following spontaneous fission and (alpha,n) reactions. Rn outgassing from materials and subsequent decay of daughters as well as their plate-out onto detector material surfaces also presents background that can limit experimental sensitivity. There is a wide range of techniques used to measure and quantify such radioactivity within, and being released from materials, with each sensitive to different types of radiation, over different energy ranges, and at differing sensitivities. Specifically, gamma-ray spectroscopy, mass spectrometry, direct radon emanation measurements systems and alpha-particle spectroscopy, when used together and with sufficient sensitivity, can accurately characterise radioactive emission from construction materials and be used to accurately build up its background model. The UCL Dark Matter Group has developed low-background capability in the UK to establish world-leading facilities that cover the entirety of these complementary techniques. These facilities are managed by UCL and partners at the Boulby Underground Laboratory, Rutherford Appleton Laboratory, University of Edinburgh.
BUGS @ Boulby Mine
The Boulby Underground Germanium Suite (BUGS) comprises of an array of low-background, High-Purity Germanium (HPGe) detectors operating in a dedicated ISO Class 6 cleanroom within the Boulby Underground Laboratory, located 1.1 km underground in the north-east of England, UK. Established initially to perform material radio-assays for the construction of the LUX-ZEPLIN dark matter experiment, BUGS has since been extended to 6 ultra-low background gamma spectroscopy detectors that provide high-throughput and high-sensitivity assays servicing the international rare-event search community. BUGS employs a wide range of detectors to meet the diverse material sample types and specific isotopic sensitivities required for low-background experiments, including: large-mass and high-efficiency p-type HPGe; low-threshold planar Broad Energy Germanium (BEGe); and well-type Small Anode Germanium (SAGe). Each detector is housed in shielding castles developed in collaboration with Lead Shield Engineering Ltd, UK. The bespoke shields are constructed using 9 cm thickness of lead outside 9 cm thick OFHC copper. The copper and lead come from Boulby stock material that has remained underground for at least 2 decades, and selected for the BUGS castles after qualitative assay of batches to determine those with the lowest radioactivity. The internal cavity of each castle is of dimensions (200 x 220 x 510) mm3 and is continuously purged with N2 fed through a meandering bore to reduce the already low ambient level of 3 Bq/m3 of 222Rn at Boulby to negligible levels. LN2 filling and N2 operations are entirely automated and use gas extracted from the low-Rn underground laboratory itself. Monitoring and custom analysis tools deliver assay results from any sample geometry or configuration from each of the detectors that have been extensively cross-calibrated with radioactive sources and against other detectors in the UK and internationally, as well as against high sensitivity mass-spectrometry systems. The 6 BUGS detectors, most of which can be seen in the image, below, are named after former mines in the Boulby area: Belmont, Merrybent, Lunehead, Chaloner, Roseberry, and Lumpsey.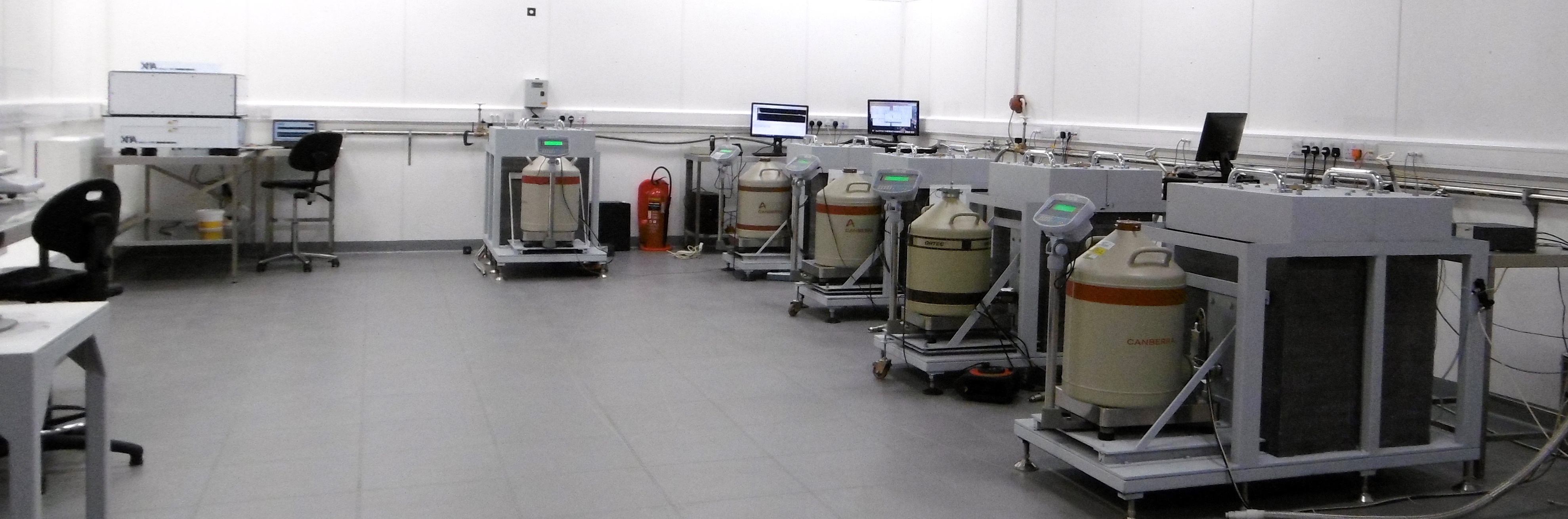
Belmont is a 600 cc p-type coaxial high-purity germanium detector. A number of the materials used to construct the cryostat for this detector were assayed using existing BUGS germanium detectors in order to ensure as low a background as possible. Due to its size Belmont has the potential to assay samples with a precision and sensitivity superior to the other detectors. Merrybent is a 375 cc p-type coaxial high-purity germanium detector. Lunehead is a 2.0 kg Ortec GEM-XX240-S standard p-type coaxial detector with a nominal relative efficiency of 92%. Chaloner is a 0.8 kg BE5030 Broad Energy Germa- nium (BEGe). This detector has a nominal front face surface area of 50 cm2 and a length of 30 mm. The BEGe detector is constructed in a planar format and has a small inner electrode for signal output. The geometry of this detector allows for an effectively dead-layer free front face which gives excellent efficiency for low-energy gamma-rays such as the 46.5 keV line associated with the beta-decay of 210Pb to 210Bi in the 238U series. Roseberry is a Mirion BEGe BE6530 detector. This larger surface planar BEGe detector has a 65 cm2 area on its front face and a 30 mm thickness. Lumpsey is a 1.5 kg Mirion GSW275L small anode germanium (SAGe) well-type detector with a nominal minimum detector volume of 275 cm3 and a well diameter and depth of 28 mm and 40 mm, respectively. Lumpsey has a measured relative efficiency of 69%. The geometry of the detector allows almost complete coverage for samples screened in the well thus allowing high efficiency screening for small samples. As with the BEGe detector, the SAGe well detector allows excellent efficiency to low-energy gamma-rays. Previous generations of well detector have suffered from poor resolution but this ultra-low background SAGe well detector gives a response approaching that of the BEGe detector. The crystal is held in a stainless steel cryostat. The Lumpsey detector can be used as a standard coaxial HPGe detector for large samples placed outside the well.
ICP-MS Facility @ UCL
The University College London (UCL) ICP-MS system is housed in a dedicated ISO Class 6 cleanroom reserved for radio-assays of materials for rare-event search experiments. Initially established for the LUX-ZEPLIN construction project and deploying an Agilent 7900 ICP-MS, the facility has since been upgraded to house a state-of-the-art Agilent 8900 tandem mass triple quadrupole ICP-MS, optimised for extremely low detection limits as necessitated by current and next generation dark matter and neutrinoless double beta decay experiments. The system is fitted with an inert sample introduction kit that includes a PFA nebulizer, spray chamber and torch connector, platinum sampling and skimmer cones and a plasma torch with quartz outer body and sapphire injector. This allows up to 20% v/v acid concentration in the sample introduced to the ICP-MS, including hydrofluoric acid (HF). The limiting factor in realising reproducible high throughput sensitivity at the parts-per-trillion (ppt), g/g, level for direct measurements of 238U and 232Th is clean, standardised sample preparation requiring dedicated digestion apparatus and well developed procedures, including the use of ultra-pure acids to avoid contamination of samples. The facility has the relevant infrastructure to provide this, including: sample preparation in separate ISO Class 5 laminar flow unit (LFU), sample digestion with the Milestone ETHOS-UP closed vessel microwave digestion system with SK-15 high pressure rotor, Milestone sub-boiling point acid distillation (subCLEAN) and reflux cleaning (traceCLEAN) systems and an ELGA PURELAB flex ultra-pure de-ionised (DI) water supply (18.2 MOhm.cm, <5 parts-per-billion (ppb) total organic carbon). Finally, a Pyro-260 microwave ashing system allows for the digestion of materials such as PTFE that are resistant to most acids, including HF. The ICP-MS facility is shown in the image below, with the Agilent 8900 visible in the far left corner of the cleanroom.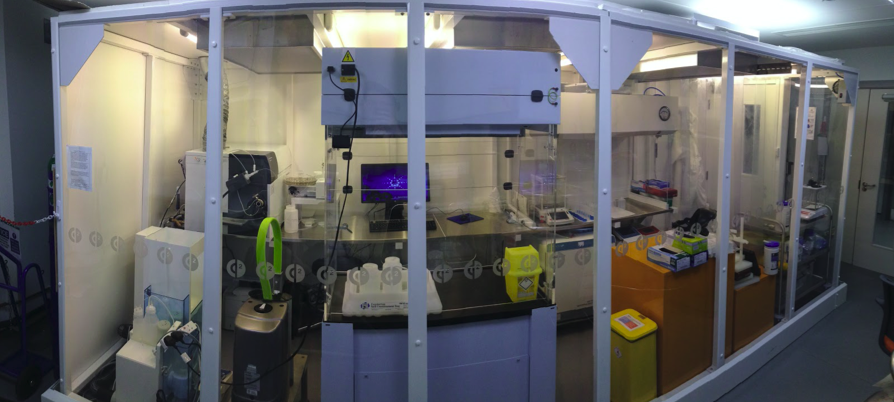
Radon Emanation @ RAL
Radon emanation from construction materials presents a major and in some cases dominant background to dark matter searches, particularly those deploying noble liquid targets where radon progeny are expected to decay uniformly across fiducial volumes. Of particular concern are events from the beta decay of 214Pb. Some fraction of radon may be removed from the dark matter targets through online purification and there is also potential to identify radon events in analysis. However, the most direct mitigation at present is to screen potential construction materials for radon directly. Residual activity from materials that are selected is also quantified through such assays, informing the experiment's background model. The UK hosts 3 radon emanation facilities used extensively for rare-event search experiments, at Boulby Mine, the Rutherford Appleton Laboratory, and UCL.The radon emanation measurement system at the Boulby Underground Laboratory is located within the ISO Class 6 BUGS cleanroom, recently installed by the University of Edinburgh. It contains a dual radon concentration line (RnCL) using activated charcoal with twin 80 litre electrostatic radon detectors using low background Si PIN diodes. These detectors, manufactured by the University of Tokyo (UoT), may be operated together or independently to increase sample assay throughput. The system incorporates 5 emanation chambers with volumes ranging from 5 to 100 litres to accommodate a range of sample sizes without unnecessarily increasing backgrounds from the emanation chamber. The RnCL operates through a 2 step process. Emanated radon atoms are firstly transferred to the RnCL via a carrier gas. It is here that the radon in the gas is captured by an activated charcoal trapping unit maintained at LN2 temperature to increase trapping efficiency, and is later heated up to release trapped radon atoms, which are transferred to the electrostatic detector. The facility achieves sensitivity to 222Rn below 0.1 mBq.
The Cryogenic Radon Emanation Facility (CREF) at the Rutherford Appleton Laboratory (RAL) and co-managed by UCL deploys a similar 80~litre Si PIN diode based detector developed by UoT, and is coupled through a radon concentration line to a series of emanation chambers, with most of these operating at ambient environmental temperature. Radon emanation assays of materials are typically performed at room temperature. However, this results in large uncertainties when translating screening results to expected rates in noble liquid targets due to the suppression of radon diffusion within and subsequent emanation out of materials. Furthermore, a component of the total emanation from nuclei recoiling out from the surface of materials, rather than through diffusion, is not expected to be affected by temperature. Accurately predicting radon emanation from materials in experiments therefore requires assays at final operating temperatures, as well as with equivalent surface treatments. The largest of the CREF emanation chambers is 200 litres in volume, is enclosed within a 500 litre cryogenic vessel, and can be cooled and stabilised at temperatures down to 77 K for measurement of radon emanation from large material samples and as a function of temperature. The CREF facility can be seen in the image below within its dedicated cleanroom located at the R5.2 building at RAL.
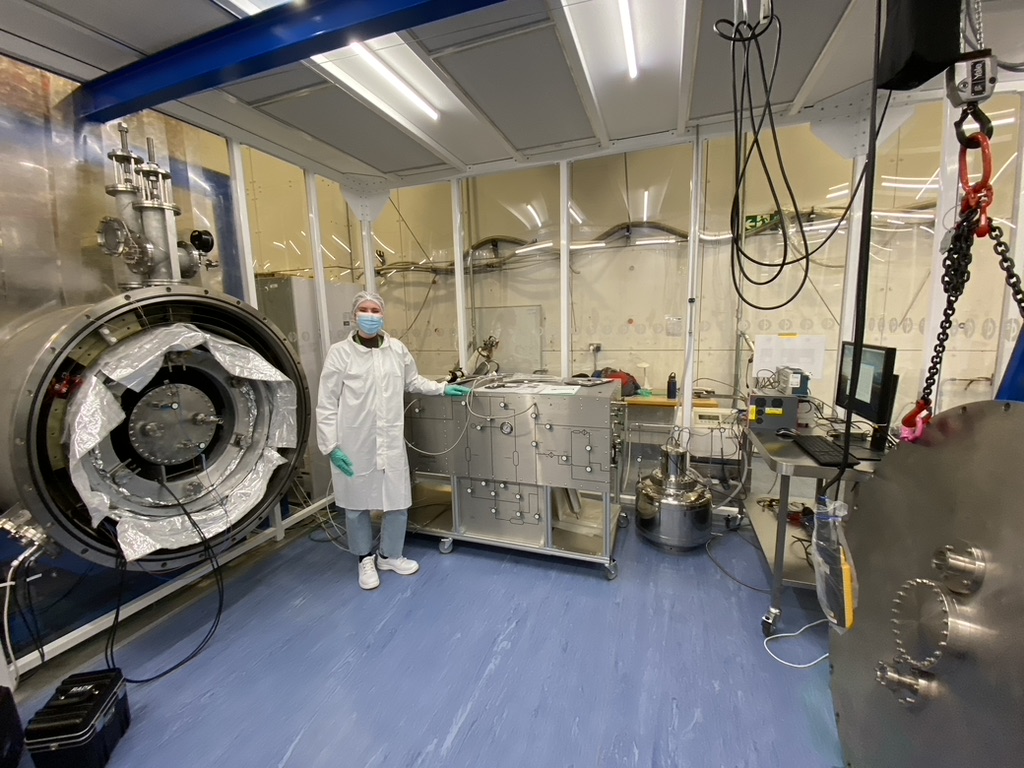
UCL operates a radon emanation system with similar Si PIN dionde detector within an 80~litre volume, developed by UoT, originally used for the SuperNEMO experiment and subsequently used extensively for the LUX-ZEPLIN experiment. It has 2 separate low-background 3 litre emanation chambers with a RnCL between chambers and detector, achieving sensitivity of 0.1 mBq to 222Rn. The designs of the Boulby radon facility and CREF are heavily based on this original system.
Alpha-screening @ Boulby Mine
The Boulby Underground Laboratory operates 2 UltraLo-1800 alpha-particle detectors, manufactured by XIA LLC, within the ISO Class 6 BUGS cleanroom. The UltraLo-1800 instruments are co-located with the gamma spectroscopy and radon emanation detectors to provide complete assay capability within the same low-radon underground facility. The overburden at 1100 m depth at Boulby delivers a factor 4 background suppression in the UltraLo-1800 alpha detectors, delivering <1 mBq/m2 sensitivity to 210Po and 210Pb on the surface of materials, as well as <30 mBq/kg sensitivity to their presence within the bulk material, complementing the capability of the BUGS BEGe detectors with direct 210Pb assays using gamma-ray emission. One of the XIA systems is shown in the image below.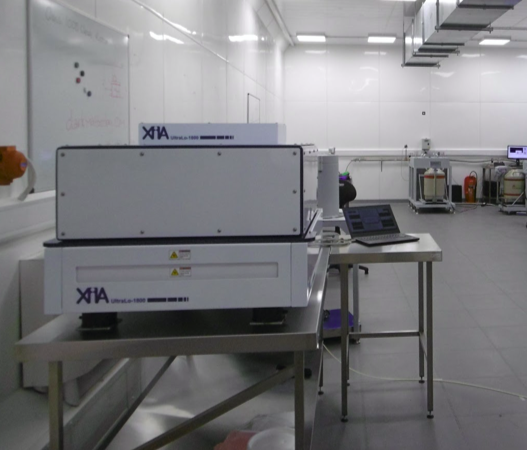